Contents
As we continue to push the boundaries of technology, power sources have become a critical area of focus. These advances have led to the evolution of traditional lithium-ion batteries to more efficient and environmentally friendly versions, such as the solid-state lithium batteries. Recently, the integration of silicon as an anode material has been attracting considerable attention in the battery technology sphere.
Why the sudden interest in silicon anodes? Well, it’s all about energy density. Silicon has an impressive theoretical charge capacity that is ten times that of the commonly used graphite. This means that batteries with silicon anodes have the potential to store a lot more energy, a feature highly desirable for applications such as electric vehicles and renewable energy storage.
However, this potential is not without its challenges. Silicon undergoes a dramatic volume change during charging and discharging, which can lead to fast degradation of the battery. The solution to this problem lies in the fields of materials science and engineering, where researchers are exploring various strategies to harness the potential of silicon anodes.
The world of solid-state lithium batteries with silicon anodes is a blend of fascinating science and promising technology. This article will take you on a journey through this exciting field, helping you understand the fundamental concepts, the potential benefits, the challenges faced, and the future prospects of this technology. So, fasten your seatbelts and get ready to delve into the world of cutting-edge battery technology—where two become one.
Understanding Lithium BatteriesÂ
Lithium batteries, particularly lithium-ion batteries, have become a staple of modern life. They power everything from our smartphones and laptops to electric vehicles and renewable energy storage systems. The reason for their widespread use is their high energy density—meaning they can store a large amount of energy relative to their size and weight.
A battery consists of three main components: an anode (negative electrode), a cathode (positive electrode), and an electrolyte that allows for the flow of ions between the two electrodes. When a battery is in use, lithium ions move from the anode to the cathode through the electrolyte, creating a flow of electrons in the external circuit that provides power to our devices. When the battery is charged, the process is reversed, with lithium ions moving from the cathode back to the anode.
In most lithium-ion batteries, the anode is made of graphite, a form of carbon. Graphite has been the material of choice due to its ability to intercalate (host) lithium ions in its layered structure during charging, and its relatively stable performance over many charge-discharge cycles.
However, the energy density of these batteries is limited by the amount of lithium ions that the graphite anode can host. And here is where silicon comes into the picture. Silicon can theoretically host much more lithium ions compared to graphite, which means it has the potential to significantly increase the energy density of the batteries. But before we delve deeper into the role of silicon, let’s first understand the function of anodes in batteries.
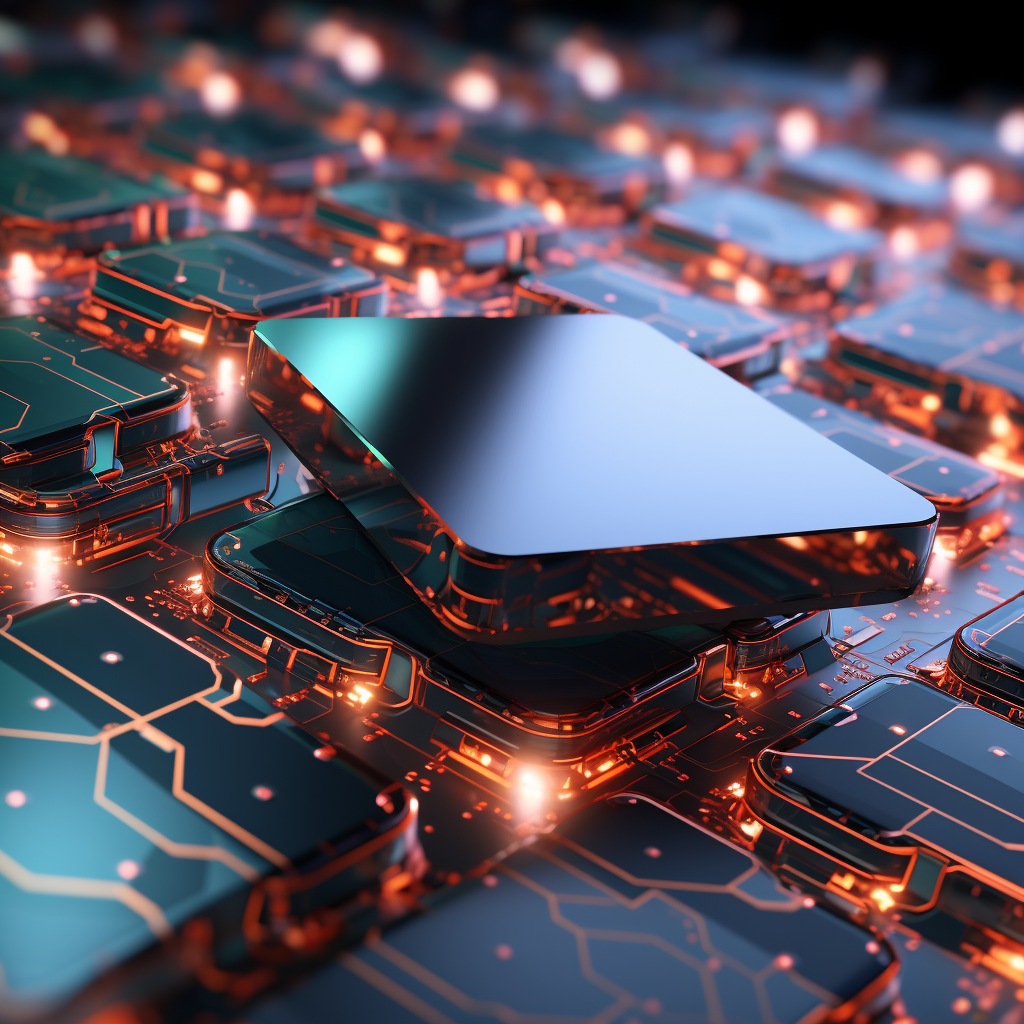
The Role of Anodes in BatteriesÂ
The anode in a battery plays a crucial role in determining the battery’s capacity, energy density, and overall performance. It’s where the action starts when you plug in your device and power it on, with lithium ions migrating from the anode through the electrolyte to the cathode. This movement of ions is simultaneously accompanied by a flow of electrons through the external circuit, providing the electric current that powers our devices.
When a battery is charged, the process is reversed: lithium ions move from the cathode through the electrolyte and are stored in the anode. The ability of an anode material to host these lithium ions, known as its charge capacity, is a key factor determining the energy density and thus the battery’s performance.
Different materials can be used for the anode, and the choice of material has a significant impact on the battery’s properties. For a long time, graphite has been the go-to anode material in lithium-ion batteries due to its good electronic conductivity, layered structure that can host lithium ions, and relatively stable performance over many charge-discharge cycles.
However, the quest for higher energy density batteries has led researchers to explore other anode materials. One of these is silicon, which has attracted considerable attention due to its high theoretical charge capacity – approximately ten times that of graphite. This means that silicon can host a lot more lithium ions compared to graphite, potentially leading to batteries with much higher energy density.
Despite these promising attributes, silicon is not without its challenges, which we will delve into in the next chapter, “Silicon as an Anode Material.” But one fact remains clear: the anode is a vital component of a battery, and the material choice for the anode can make a significant difference in the battery’s performance.
Silicon as an Anode MaterialÂ
Silicon has emerged as a promising anode material for the next generation of lithium-ion batteries due to its high theoretical charge capacity. This is the maximum amount of charge a material can theoretically hold. For silicon, this value is about ten times higher than that of graphite, the commonly used anode material.
The high charge capacity of silicon arises from its ability to host a large number of lithium ions. When a battery is charged, lithium ions from the cathode move through the electrolyte and are stored in the anode. Silicon’s atomic structure allows it to absorb a significant number of these lithium ions, leading to a high charge capacity.
However, this high charge capacity comes with challenges. One of the major ones is the significant volume expansion that silicon undergoes when it absorbs lithium ions during charging. This can lead to mechanical stress and fracturing of the silicon, causing a fast degradation of the anode and a decline in the battery’s performance over time.
Researchers have been exploring various strategies to overcome this challenge, such as designing silicon in nanostructures or combining it with other materials to accommodate the volume changes. While these strategies have shown promise, more research and development are needed to make silicon anodes practical for commercial applications.
Despite these challenges, the potential benefits of silicon anodes—namely, the significantly higher energy density—are too great to ignore. This has spurred on continued research efforts to unlock the potential of silicon anodes and bring about the next evolution in lithium battery technology.
Solid-State Lithium BatteriesÂ
As we’ve learned, lithium-ion batteries play a crucial role in our modern, technology-driven world. However, traditional lithium-ion batteries have a liquid electrolyte, which presents safety risks due to leakage or potentially hazardous reactions under certain conditions. Enter solid-state lithium batteries, an emerging technology that promises to redefine the landscape of energy storage.
Solid-state lithium batteries replace the liquid electrolyte with a solid one. This significant modification brings several advantages. First, solid-state batteries are generally safer than their liquid counterparts, largely avoiding risks of leakage or combustion. Second, they can operate at higher voltages, which can potentially lead to higher energy densities.
However, the transition from liquid to solid electrolytes is not without its challenges. One of the main ones is the interface compatibility between the solid electrolyte and the electrodes. A good connection at this interface is crucial for lithium ions to move effectively between the anode and cathode during charging and discharging.
Various types of solid electrolytes have been studied, including ceramics, polymers, and glass. Each has its strengths and weaknesses in terms of ionic conductivity, stability, and interface compatibility. Ongoing research is aimed at optimizing these properties to achieve high-performance solid-state batteries.
The solid-state design opens up new possibilities for anode materials as well. Silicon, with its high charge capacity, is a promising candidate and has been the subject of extensive research in the context of solid-state batteries.
The Integration of Silicon Anodes in Solid-State Lithium Batteries
Integrating silicon anodes into solid-state lithium batteries can potentially combine the advantages of both technologies, resulting in batteries with high energy density and improved safety. However, the successful integration of these two technologies requires addressing several challenges.
One of the main challenges is the compatibility between silicon anodes and solid electrolytes. As we’ve learned, silicon undergoes a significant volume expansion when it absorbs lithium ions during charging. This volume expansion can cause mechanical stress and degradation at the interface between the silicon anode and the solid electrolyte, leading to a decrease in battery performance over time.
Addressing this challenge requires careful engineering of the materials and the battery structure. Researchers have been exploring different strategies, such as using composite anodes that mix silicon with other materials, creating nanostructured silicon to better accommodate the volume changes, and developing solid electrolytes that can maintain good contact with the anode despite its volume changes.
Another challenge is ensuring fast and efficient transport of lithium ions between the anode and the solid electrolyte. This requires a solid electrolyte material that has high ionic conductivity and is stable at the interface with the silicon anode.
Despite these challenges, the potential benefits of integrating silicon anodes into solid-state lithium batteries—namely, significantly higher energy densities and improved safety—are driving ongoing research and development efforts.
Advantages and ChallengesÂ
The integration of silicon anodes into solid-state lithium batteries brings about a host of potential advantages, as well as significant challenges that need to be addressed.
Advantages
- Higher Energy Density: Silicon anodes can hold more lithium ions compared to traditional graphite anodes, potentially leading to a significant increase in energy density. This could translate into longer battery life for devices or increased range for electric vehicles.
- Improved Safety: Solid-state batteries eliminate the liquid electrolyte, which is a common cause of battery leakage and thermal runaway. This could improve the safety of batteries in a variety of applications.
- Longer Lifespan: Solid-state batteries have the potential to offer longer lifespans. The solid electrolyte can be more resistant to degradation compared to liquid ones.
Challenges
- Volume Expansion: The significant volume expansion of silicon during charging can cause mechanical stress and degradation of the anode and the interface with the solid electrolyte.
- Interface Compatibility: Ensuring good contact between the solid electrolyte and the silicon anode is crucial for efficient operation of the battery. This can be challenging due to the volume changes of silicon and the rigid nature of most solid electrolytes.
- Ionic Conductivity: The solid electrolyte needs to have high ionic conductivity to allow for efficient transport of lithium ions. However, many solid electrolytes have lower ionic conductivity compared to liquid ones.
The potential benefits of integrating silicon anodes into solid-state lithium batteries are driving ongoing research and development efforts to overcome these challenges. If successful, this could lead to a new generation of batteries with higher energy densities and improved safety, revolutionizing a wide range of industries from consumer electronics to electric vehicles and renewable energy storage.
The Future of Silicon Anodes and Solid-State Batteries
The integration of silicon anodes into solid-state batteries holds great promise for the future of energy storage technology. If the challenges associated with this integration can be successfully overcome, we could see a new generation of batteries with higher energy densities, longer lifespans, and improved safety.
The potential applications of these advanced batteries are vast. Longer-lasting batteries could significantly enhance the user experience for smartphones, laptops, and other portable electronics. Higher energy densities could extend the range of electric vehicles and make them more competitive with their gasoline-powered counterparts. Improved safety could be especially beneficial for large-scale energy storage systems, reducing the risk of fires and other accidents.
Furthermore, the development of these advanced batteries could have a significant impact on renewable energy adoption. One of the key challenges with renewable energy sources like wind and solar is their intermittency—they produce power when the wind is blowing or the sun is shining, but not at other times. High-capacity, long-lasting batteries could store the energy produced during peak production periods and release it when needed, making renewable energy more reliable and practical.
However, it’s important to remember that the successful development of silicon-anode, solid-state batteries is not guaranteed. It requires overcoming significant technical challenges, and there’s always the possibility that other battery technologies could prove more viable.
Regardless of the specific technologies that prevail, the future of energy storage looks very promising indeed. With continued research and innovation, we can expect to see significant advancements in battery technology in the years to come, driving progress in a wide range of industries and helping us transition to a more sustainable energy future.